Grape phylloxera (Daktulosphaira vitifoliae) were first detected in France in the middle of the 1860s. They were soon noticed across Europe and eventually devastated most of Europe’s vineyards.1 Their rapid dispersal suggests that they were widely spread before being noticed, likely on infested rootings from growers and nurseries, and on contaminated equipment. The phylloxera crisis was preceded by the inadvertent introduction of the grape mildew diseases. Phylloxera and the mildews are North American in origin and their introduction into Europe was tied to interest in the North American grape species, which were being grown as horticultural curiosities and had attracted attention due to their strong mildew resistance. However, their fruit and wine quality is very different from Vitis vinifera. The mildew diseases were soon controlled with fungicides composed of sulfur and copper. Attempts to control phylloxera first utilized gaseous forms of carbon bisulphide, which ultimately was ineffective. Grape breeders and nurseries then began hybridizing V. vinifera cultivars with wild American Vitis species to create the hybrid direct producers.
The goal of these breeding efforts was to produce a grapevine with high quality fruit and wine, and at the same time a root system with strong resistance to phylloxera feeding. Unfortunately, these efforts yielded cultivars with subpar fruit and wine quality when compared to V. vinifera, and only moderate levels of phylloxera resistance. However, they were often very resistant to powdery and downy mildew, and had relatively strong winter cold tolerance. They are still grown in some areas with very cold winters, but their wines tend to be very acidic with herbaceous, peppery characters, as well as blue-purple coloration and fox-den aromas.
“The final and successful approach to control phylloxera was the use of resistant rootstocks upon which V. vinifera cultivars could be grafted.”
The final and successful approach to control phylloxera was the use of resistant rootstocks upon which V. vinifera cultivars could be grafted. It was reasoned that phylloxera resistance should be present in the North American Vitis species that co-evolved with this aphid-like pest. There are about 30 Vitis species in North America,2 and only two of them root easily from hardwood cuttings, V. riparia and V. rupestris. These two species have strong phylloxera resistance, but differ in a number of characteristics. Vitis riparia grows in most fine-textured, often alluvial soils and has a shallow and primarily fibrous root system. It has the widest distribution of any North American Vitis species and is found from the Rocky Mountains to the Atlantic coast and from Canada to Texas. Forms of this species have excellent winter cold tolerance. It produces a relatively vigorous vine climbing to 15 meters. Vitis rupestris grows in creek beds of coarse gravel caused by periodic and highly erosive flooding. To survive in these creek beds it has deep and relatively thick root system for anchorage. It is a shrubby plant to about 1 m and rarely climbs in trees. It used to be found from Pennsylvania to Texas, but the westward movement of Americans and their cattle in the mid 1800s pushed this species to extinction. It is now only common in southern Missouri, most often in creeks at the base of steep ravines where it is protected from cattle.
These two species were successfully used as rootstocks to replant vineyards that had failed to phylloxera. At first the replanted vineyards grew well, but when vineyards were planted on calcareous soils, these grafted vineyards began to fail to lime-induced chlorosis. French botanists and breeders sought out T.V. Munson, a grape breeder and the US expert on North America Vitis, who suggested the use V. berlandieri from the limestone hills of central Texas (he later received the French Legion of Honor Chevalier du Mérite Agricole for his efforts). This species possess strong lime tolerance and is phylloxera resistant, but it roots poorly from hardwood cuttings. Thus, V. berlandieri was hybridized with the two species that root well: V. riparia to produce rootstocks with lime tolerance, lower vigor and better adaptation to cooler climates; and V. rupestris to produce lime tolerant rootstocks with higher vigor and better adaptation to warmer, drier climates.
“Logic suggests that we should strive for greater genetic diversity to help prevent the selection of more aggressive pests and pathogens.”
Hundreds of rootstocks were created by the 1920s and we entered a period of about 70 years where rootstock breeding received relatively scant attention. The traditional rootstocks have been very successful, but there are a number of factors that have prompted renewed interest in rootstock breeding. The first of these is agricultural practice. Throughout most of the World, grape is considered to be a high value crop, and vineyard land devoted to grape is valuable and seldom used for other crops. Yet, one of the most important agricultural principles is the need to rotate crops on a given site to prevent the development and build up of aggressive pathogens and pests. This can be done in vineyards by altering rootstock choices when replanting. However, there are relatively few rootstocks being used, and alternative choices based on soils, wine quality and tradition are limited. There are other factors that also support the need for greater rootstock breeding efforts. First is the relatively narrow genetic base of the rootstocks being using. Recently completed genetic analysis of the world’s most commonly used rootstocks show them to be very closely related and that the same accessions of V. riparia, V. rupestris and V. berlandieri were used in the original hybridization efforts (Riaz, Walker in preparation). Logic suggests that we should strive for greater genetic diversity to help prevent the selection of more aggressive pests and pathogens. Foremost amongst these are phylloxera and nematodes. Moreover, the traditional rootstocks may not possess the characteristics we need to confront climate change as some viticultural regions becoming drier and more saline, and other regions will be in need of rootstocks to hasten or perhaps delay maturity. There are many other past and current rootstock needs that need to be addressed such as resistance to multiple nematode species, better resistance to fanleaf degeneration, tolerance to expression of the red leaf virus diseases, better salinity and drought tolerance.
The rootstock breeding program at the University of California, Davis has been underway since 1989. It follows on the nematode and fanleaf resistance breeding efforts of L.A. Lider, and H.P. Olmo before him.3,4 The program’s breeding objectives are broad and durable nematode resistance, a better understanding of phylloxera resistance, tolerance to fanleaf degeneration and the red leaf virus complex, tolerance to salinity and drought, and combinations of these traits. The first rootstocks to be patented and released from these efforts were GRN-1, GRN-2, GRN-3, GRN-4 and GRN-5 (Grape Rootstocks for Nematodes), and they were released in 2009 and 2010.5 They are resistant to a common strain of root-knot nematode (Meloidogyne incognita) that feeds on V. vinifera and many rootstocks, and two strains that overcome Freedom and Harmony rootstocks (one of which is M. arenaria, HarmA and the other M. incognita, HarmC). They also resist Xiphinema index (the dagger nematode vector of fanleaf degeneration), all of these nematodes in a combined inoculum, and they resist this combined inoculum of nematodes at 30°C soil temperature, at which resistance based on V champinii – Harmony, Freedom, Ramsey and Dog Ridge – breaks down. These soil temperatures are common in the upper soil profile of warm climate vineyards, the same zone that contains the greatest root proliferation and largest populations of soil-borne pests. Thus, these soil temperatures have the potential to encourage the evolution of more aggressive nematode populations. The GRN series rootstocks also resist phylloxera, lesion nematode, four of them resist citrus nematode, and one, GRN-1, resists all these as well as ring nematode (Table 1).5 The nematode resistance in GRN-2 through GRN-5 comes from forms of V. champinii V. riparia and V. rufotomentosa. GRN-1’s resistance comes from Muscadinia rotundifolia. To achieve the highest rooting and grafting success with GRN-1 and GRN-5 only well-lignified mature canes should be used. GRN-1 should also be carefully established in the vineyard. If vines have active growth when late Fall or early Winter freezes occur, they can die back to the trunk.
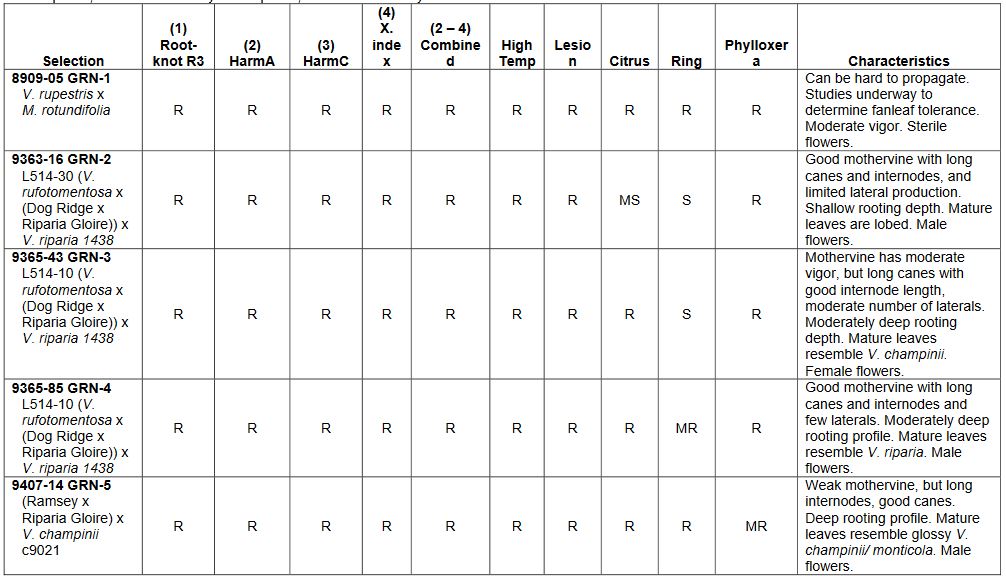
The GRN series rootstocks are slowly gaining acceptance. Most are moderately vigorous and are thus far tolerating high and diverse populations of nematodes in vineyards across California. These rootstocks have also been used in crosses to combine broad nematode resistance with salt tolerance. GRN-1 has not been used as a parent because it is sterile with 39 chromosomes, as in the case of almost all Vitis x Muscadinia hybrids. The grape breeding program is using several rare fertile V. vinifera x M. rotundifolia hybrids produced by H.P. Olmo, but their phylloxera resistance is being carefully evaluated prior to use as parents. Muscadinia rotundifolia has exceptional resistance to soil-borne pests and is the source of fanleaf degeneration resistance in UC Davis’ O39-16.3 This V. vinifera x M. rotundifolia (VR) hybrid is now off patent restrictions, but is sterile. There were concerns about O39-16’s ability to resist phylloxera given its 50% V. vinifera parentage, but it has proven to be phylloxera resistant for over 30 years of vineyard use. O39-16 is also one of our best sources of ring nematode resistance. However, it is susceptible to root-knot nematodes and is not well adapted to calcareous soils.
Grapevines are sensitive to salty water and soils. The damage is not from high levels of sodium, to which they are relatively tolerant, but from chloride. The Walker lab has worked to develop better methods to screen for chloride tolerance in grape and to better understand the genetics of salt tolerance. Although the rootstocks Ramsey and 140Ru have been used successfully in saline soils, as climates get drier better forms of chloride tolerance or exclusion will be needed. We have amassed an extensive collection of southwestern Vitis species over the last 25 years. These collection efforts were stimulated by the discovery that forms of V. arizonica from northern Mexico were the inadvertent parents in a number of crosses made by H.P Olmo.6 These crosses were intended to be V. rupestris x M. rotundifolia but many forms of V. arizonica contaminated the crosses. This was fortuitous as within the mixed crosses we found resistance to Xiphinema index, root-knot nematode, phylloxera and Pierce’s disease (PD). Individuals from these populations have been used as parents in our PD resistant winegrape breeding and in our rootstock breeding.
Over 700 accessions of southwestern Vitis have been collected from trips throughout California, Nevada, Arizona, Utah, Colorado, Texas, and Oklahoma. We have found exceptional forms of chloride tolerance in V. acerifolia and V. doaniana from saline banks of the Red River between Oklahoma and Texas, and in V. girdiana from desert springs in Nevada and Arizona. We have crossed the salt resistant accessions with the GRN series and other rootstocks to combine nematode resistance and chloride tolerance and are currently selecting for the combination of these traits as well as different levels of vigor and root architecture. One of the more interesting traits available in this germplasm is strong resistance to Xiphinema index, given that this nematode is considered to be from Central Asia and North American Vitis spp. would not be expected to resist it. We have genetically and physically mapped this resistance and named the resistance locus XiR1.7,8 This locus was found in a form of V. arizonica from Loreto, Mexico, and has since been found in other forms of V. arizonica. We have transformed XiR1 into Rupestris St. George to prove its function and are using tightly linked genetic markers to combine X. index resistance with other traits through marker-assisted selection.
Strong sources of boron tolerance were also found in the southwestern Vitis germplasm: in V. girdiana from near boron deposits in southeastern California; V. arizonica from alkali flats in southern Arizona; and V. acerifolia from northern Texas. We are incorporating this resistance into our salt and nematode resistant rootstocks and have developed mapping populations to further characterize this trait.
Finally, resistance to Xylella fastidiosa, the causal agent of Pierce’s disease, is also common in the southwestern Vitis species.9,10 We have genetically and physically mapped resistance from a form of V. arizonica from Monterrey (Mexico) and continue to evaluate and genetically map PD resistance from various forms of V. arizonica collected from Arizona, New Mexico and Texas.
One of the most interesting aspects regarding the southwestern US Vitis is why they have not been used in rootstock breeding. In fact, until recently there was only one accession of V. arizonica in the United States Department of Agriculture’s National Germplasm Repository for Grape, and very poor characterization of the Vitis species from west of Texas. These species root relatively poorly from hardwood cuttings, and some tend to be relatively brushy in growth habit leading to limited yields of cuttings. Additionally, there were concerns that their phylloxera resistance was insufficient given that the root-feeding forms of phylloxera are not common across the southwestern US. Although, foliar feeding forms are common when Vitis spp. are found near streams and creek beds that are protected by tree canopies. Finally, the Vitis species in the dry areas of the southwestern US have very patchy distributions, and far more effort is required to observe and collect them than for Vitis species in the wetter eastern US. The southwestern Vitis spp. are poorly documented and are in fact complexes and intergrading hybrids among V. arizonica, V. girdiana, V. acerifolia, V. riparia, and perhaps V. monticola, V. berlandieri, V. candicans, and V. rupestris. Fortunately, this germplasm exists and as it is better understood and characterized it will play a large role in the breeding of rootstocks capable of addressing climate change and evolving soil-borne pests.
References
1. Granett, J.; Walker, M.A; Kocsis, L; Omer, A.D: Biology and management of grape phylloxera. Annual Review of Entomology 2001; 46: 387-412.
2. Walker, M.A.: The Genus Vitis, Its Species and Its Rootstocks. IN: Compendium of Grape Diseases, Disorders, and Pests, 2nd Edition, Ed. W.F. Wilcox, W.D Gubler and J.K. Uyemoto et al. American Phytopathology Press, 2015.
3. Walker, M.A.; Lider, L.A.; Goheen, A.C.; Olmo, H.P.: VR O39-16. HortScience 1991; 26: 1224-5.
4. Walker, M.A.; Wolpert, J.A.; Weber, E.: Field screening of grape rootstock selections for resistance to fanleaf degeneration. Plant Disease 1994; 78: 134-6.
5. Ferris, H.; Zheng, L.; Walker, M.A.: Resistance of grape rootstocks to plant-parasitic nematodes. Journal of Nematology 2012; 44:377-86.
6. Riaz, S.; Vezzulli, S.; Harbertson, E.S.; Walker, M.: Use of molecular markers to correct grape breeding errors and determine the identity of novel sources of resistance to Xiphinema index and Pierce’s disease. American Journal of Enology and Viticulture 2007; 58: 494-8.
7. Xu, K., Riaz, S.; Roncoroni, N.C.; Jin, Y,; Hu, R.; Zhou, R.; Walker, M.A.: Genetic and QTL analysis of resistance to Xiphinema index in a grapevine cross. Theoretical and Applied Genetics 2008; 116: 305-11.
8. Hwang, C-F., K. Xu, R. Hu, R. Zhou, S. Riaz and M.A. Walker. Cloning and characterization of XiR1, a locus responsible for dagger nematode resistance in grape. Theoretical and Applied Genetics (2010) 121: 789-99.
9. Krivanek, A.F., Riaz, Walker, M.A.: 2006. The identification of PdR1, a primary resistance gene to Pierce’s disease in Vitis. Theoretical and Applied Genetics 2008; 112: 1125-31.
10. Riaz, S., Tenscher, A.C.; Rubin, J.; Graziani, R.; Pao, S.S.; Walker, M.A.: Fine-scale genetic mapping of two Pierce’s disease resistance loci and a major segregation distortion region on chromosome 14 of grape. Theoretical and Applied Genetics 2008; 117: 671-81.